Quantum Computing
Electron Movement Capture Helps Maximize Traditional and Quantum Computing
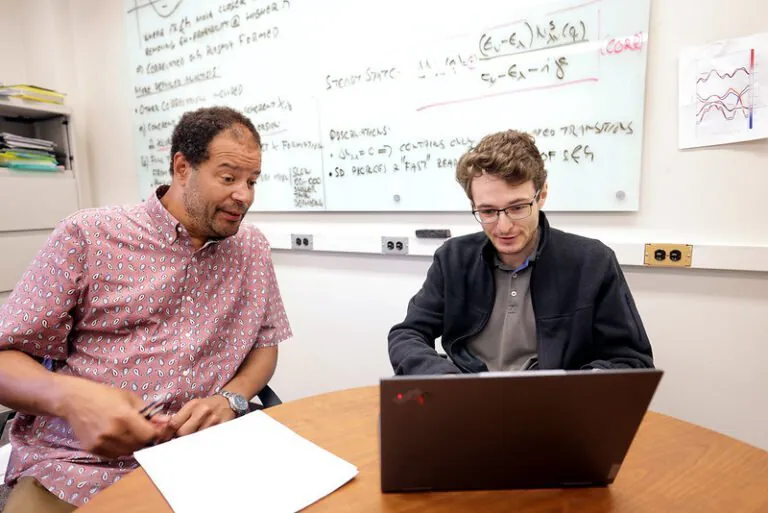
A team of researchers from the University of Michigan and the University of Regensburg have captured electron movement at the fastest speed to date. The team captured it in attoseconds, and this new development could help maximize traditional or quantum computing speeds. The research provides new insight into how electrons behave in solids.
The study was published in Nature.
Increasing Processing Speeds
By seeing electrons move in these small increments, which are one quintillionth of a second, experts could increase processing speeds up to a billion times faster than current capabilities.
Mackilo Kira, who led the theoretical aspects of the study, is a U-M professor of electrical engineering and computer science.
“Your current computer’s processor operates in gigahertz, that’s one billionth of a second per operation,” Kira said. “In quantum computing, that’s extremely slow because electrons within a computer chip collide trillions of times a second and each collision terminates the quantum computing cycle.”
“What we’ve needed, in order to push performance forward, are snapshots of that electron movement that are a billion times faster. And now we have it.”
According to Rupert Huber, who is a professor of physics at the University of Regensburg and corresponding author of the study, the results could greatly impact the field of many-body physics, even more so than computing.
Huber led the study.
“Many-body interactions are the microscopic driving forces behind the most coveted properties of solids — ranging from optical and electronic feats to intriguing phase transitions — but they have been notoriously difficult to access,” Huber said. “Our solid-state attoclock could become a real game changer, allowing us to design novel quantum materials with more precisely tailored properties and help develop new materials platforms for future quantum information technology.”
Observing Electron Movement
Researchers have traditionally relied on short bursts of focused extreme ultraviolet (XUV) light to see electron movement within two-dimensional quantum materials. The XUV bursts reveal the activity of electrons attached to an atom’s nucleus. However, the large amount of energy carried in the bursts makes it difficult for clear observation of the electrons that travel through semiconductors, which is the case in current computers and materials being explored for quantum computing.
To overcome these challenges, the team first employed two light pulses with energy scales that match those of the movable semiconductor electrons. The first pulse was infrared light, which puts the electrons into a state that enables them to travel through the material. The second pulse was a lower-energy terahertz pulse, which forces the electrons into controlled head-on collision trajectories. When the electrons crash, they produce bursts of light, which reveals interactions behind quantum information and exotic quantum materials.
“We used two pulses — one that is energetically matched with the state of the electron, and then a second pulse that causes the state to change,” Kira explained. “We can essentially film how these two pulses change the electron’s quantum state and then express that as a function of time.”
This new sequence developed by the time enables time measurement with high precision.
“This is really unique and took us many years of development,” Huber said. “It is quite unexpected that such high-precision measurements are even possible if you remember how ridiculously short a single oscillation cycle of light is — and our time resolution is one hundred times faster yet.”
Quantum computing can solve countless problems that are too complex for traditional computing, and advancements in quantum capabilities could lead to many solutions.
Markus Borsch is a U-M doctoral student in electrical and computer engineering and co-author of the study.
“No one has been able to build a scalable and fault-tolerant quantum computer so far and we don’t even know what that would look like,” Borsch said. “But basic research like studying how electronic motion in solids works on the most fundamental levels might give us an idea that leads us in the right direction.”
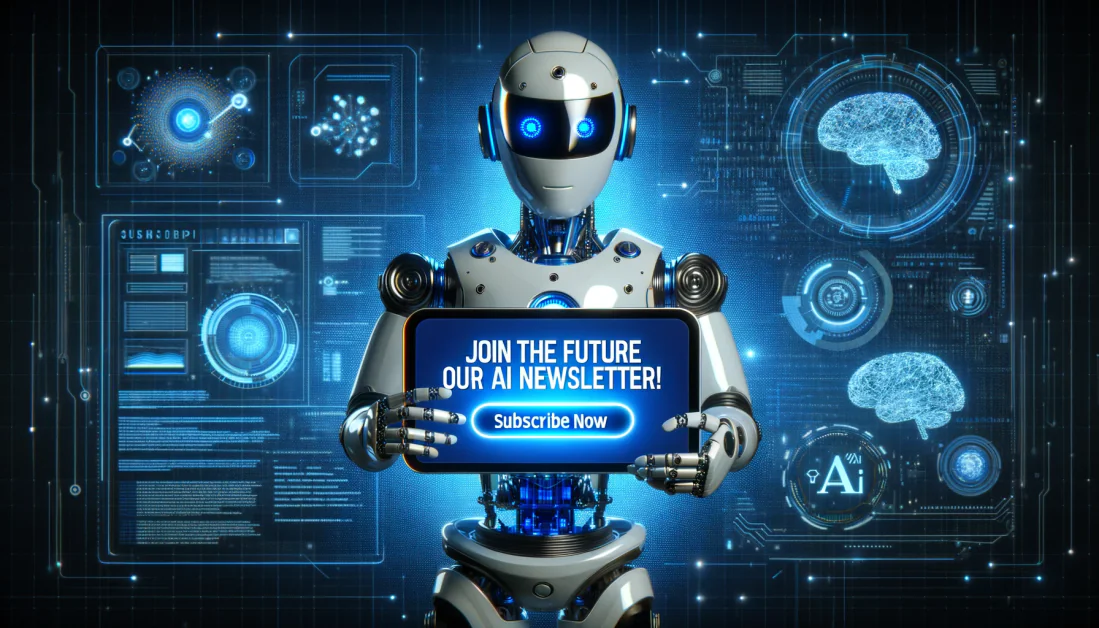
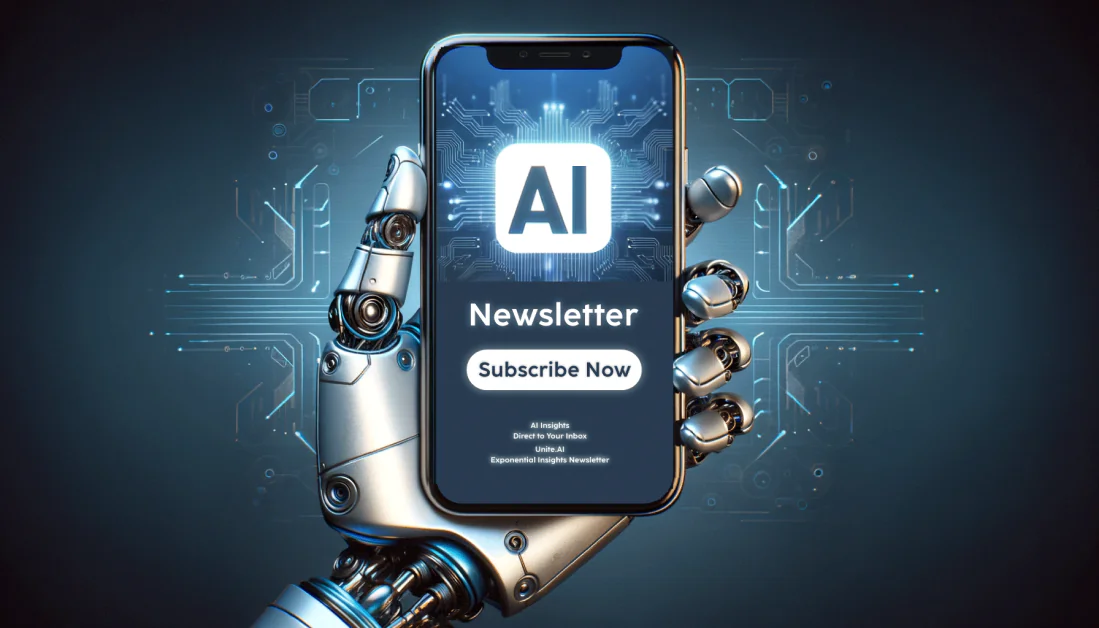